X-rays of the Earth: Unlocking the secrets of the planet
Have you ever stopped to think about how we may know the composition and structure of the Earth’s interior? As much as Jules Verne made us dream of a trip to the center of our beloved planet, it is only a fantasy. The truth is that most of the data we have about the interior of the Earth (no matter how deep) have been collected indirectly. In the same way that doctors can take images of the inside of our bodies with scanners and ultrasound scans, scientists dedicated to earth sciences have developed technologies that allow us to see the bowels of our planet.
But beyond the scientific curiosity to understand the planet we live on and its internal dynamics, being able to visualize the interior of the Earth has been a milestone for the development of society. Let me explain. The Earth’s crust is the outermost layer of the Earth, with a thickness of 15 to 20 km, depending on where we are. The rocks that compose it are a source of various resources: minerals to create batteries, metals to produce steel, drinking water accumulated in aquifers, natural gas, or oil. In addition, the deeper we are, the hotter the rocks are, which we can use to heat our homes with geothermal energy.
To take advantage of all these resources, it is necessary to detect where they are located, the type of geological structure in which they are housed, the shape of the deposits, and the behavior of the rocks that host the resources, among other things. And this is why it is essential to be able to take these “pictures” of the subsurface.
The most commonly used method to take these indirect photos is refraction seismic. A word that you will forget by the time you finish reading this blog (I don’t blame you), so I’d better explain what it consists of: in a nutshell, it consists of generating seismic waves at the surface or shallow depth, placing receivers in the area and detecting the trajectory of the waves through the crustal layers. Thanks to physics, we know that the waves change their trajectory when they reach places where the properties of the rocks change, just like when you put a pencil in a glass full of water, and the pencil seems to change shape. With these mini-earthquakes, which are not perceptible to humans, we obtain the trajectories of thousands of waves, whose reconstruction gives us an idea of what kind of structures are in the subsoil.
Here, we run into a significant problem: we cannot be 100% sure that our interpretations are correct like any indirect measurement. We do not know if the rock properties we have inferred are valid, if we have lateral changes, or if any of the algorithms and conditions we have applied to reconstruct the data are wrong. To top it off, the resolution of our measurements is limited.
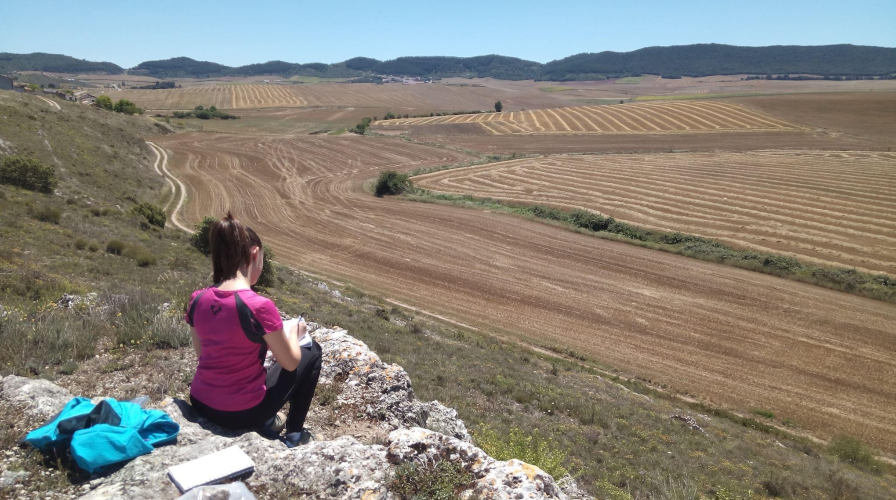
Towards a clearer subsurface: Technological innovation for a sustainable energy transition
The resolution of the images we had until now was sufficient for energy companies to decide whether to exploit gas or oil fields. But society is changing. We now have to limit the exploitation of fossil resources and promote energy production in a greener way. In this energy transition, we are going to have to use the subsoil either to generate geothermal energy, inject atmospheric CO2 into the subsoil rocks, or store excess solar/wind energy during peak production. These projects are not economically attractive because we need to know the subsurface structures in detail to develop them safely and sustainably. This means designing fast and relatively inexpensive strategies that help us to reduce uncertainty in the subsurface images.
Today, subsurface models apply several limiting simplifications, including very little detail on rock characteristics. In my research group, we think that we must avoid simplifications and investigate the connection between changes occurring at small scales and their impact on the wave trajectory to improve interpretations. In this way, we can study whether observations classified as “noise” are actually due to changes in the geology.
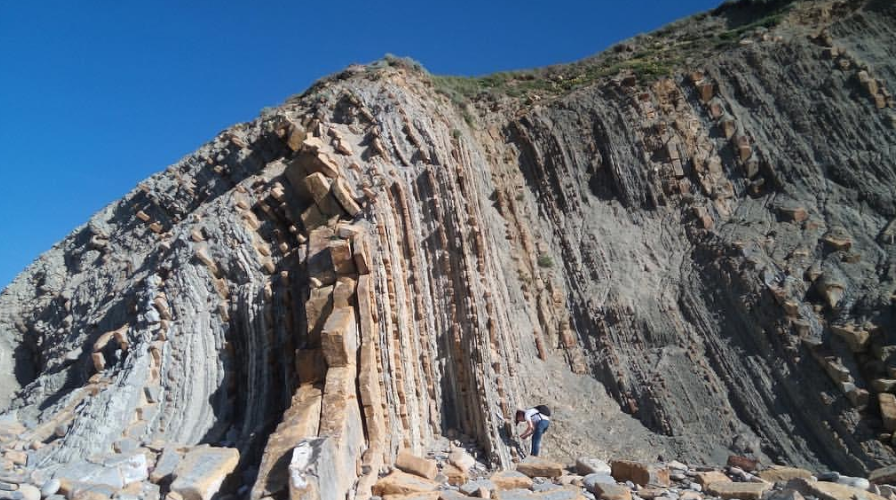
The best way to approach this idea is to use our existing geological knowledge. For example, we know that the subsurface is organized in layers. These layers can be flat, inclined, formed by different types of rocks, folded, or fractured. Any of these geological structures that we can find in the subsurface result from past geological processes (still active on the earth’s surface and that we know how they work).
So far, we have demonstrated a noticeable impact on the images when we apply the strategies we are developing. The images we generate allow us to recognize structures that were previously overlooked. The next step is to demonstrate that our method can be applied and that we can extract more detailed interpretations of the subsurface by combining it with machine learning techniques. And, therefore, to reduce uncertainty.
Our goal in developing these protocols is to reduce uncertainty and, consequently, to make energy transition projects more profitable. A technology capable of making the energy sector greener. It all sounds nice and promising, but there is a dark side. This technology could make the production of natural gas and oil fields that are not active today more profitable because they are too uncertain. It’s a double-edged sword. I know, and society often reminds you of it. However, many of our technologies, from a hammer to a GPS, can be used to harm, yet they remain indispensable to the progress of humanity.
Can you help us grow? Become a member and participate. Spread the word on the networks. Contact us and tell us about yourself and your project.
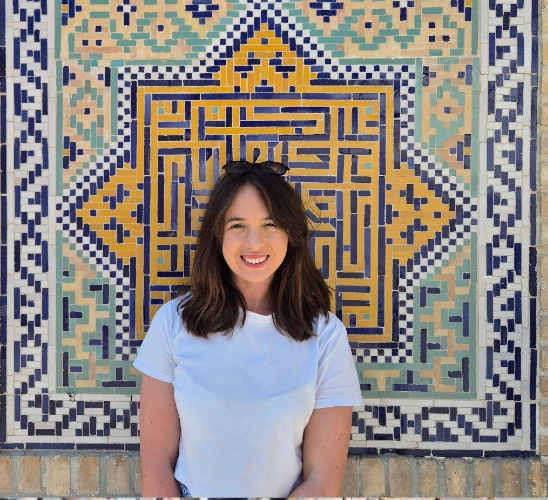
Andrea Cuesta Cano
PhD student at TU Delft
At CENL, I am in charge of the Twitter/X account, and sometimes, I collaborate with others to develop infographics about geology. In my free time, I like to watch TV series, play Rummikub or a video game, discuss politics, and see new places (although I have few places left in the Netherlands).